EV Battery Replacement Costs: A Technical Deep Dive
As the first wave of electric vehicles reaches the end of warranty periods in 2024, owners face significant battery replacement costs, with some luxury models requiring investments comparable to new vehicle prices. This has sparked discussions about battery longevity and technological solutions.
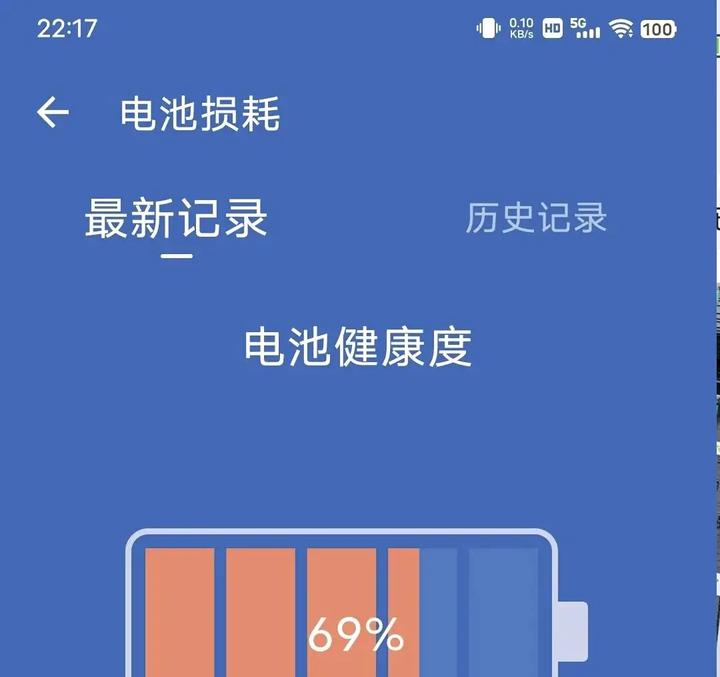
The electric vehicle (EV) industry has reached a critical juncture as the first generation of mass-market EVs approaches their battery warranty expiration. The situation has highlighted a pressing challenge: the high cost of battery replacement, which in some cases rivals the price of a new vehicle. For instance, replacing the battery pack in a Tesla Model S can cost up to $200,000, exceeding the residual value of the vehicle.
The fundamental challenge lies in battery degradation mechanisms, which occur through multiple pathways. At the microscopic level, lithium-ion batteries face several key degradation factors:
The formation and evolution of the Solid Electrolyte Interphase (SEI) layer significantly impacts battery longevity. This microscopically thin film plays a crucial role in battery performance but grows thicker over time, increasing internal resistance and reducing capacity.
The structural transformation of cathode materials during charging cycles creates mechanical stress. High-nickel cathodes, commonly used in modern EVs for their high energy density, are particularly susceptible to crystal structure changes that can lead to microcracks and capacity loss.
Cross-talk between electrodes presents another challenge. The dissolution of transition metal ions from the cathode and their subsequent deposition on the anode can accelerate degradation. This process becomes more pronounced as batteries age.
Manufacturers have implemented various technological solutions to address these challenges:
Advanced coating technologies protect electrode surfaces, helping to stabilize the SEI layer and reduce unwanted side reactions. Some companies employ artificial SEI formation techniques using carbon-based materials or fast-ion conductors.
Structural engineering approaches aim to minimize mechanical stress during cycling. Gradient-porosity structures and mechanical constraint layers help accommodate volume changes without catastrophic failure.
Novel electrolyte formulations reduce parasitic reactions and enhance stability. Localized high-concentration electrolytes show promise in extending battery life while maintaining fast-charging capabilities.
Looking ahead, solid-state batteries represent a potential breakthrough technology. These batteries promise longer lifespan and improved safety, though commercialization challenges remain. Research continues into other innovative solutions, including self-healing materials and advanced battery management systems.
For EV owners, battery degradation prediction has become crucial. Modern diagnostic tools combine physics-based models with machine learning algorithms to forecast battery health more accurately. This helps in maintenance planning and potentially extends battery life through optimized usage patterns.
The industry’s response includes new warranty policies and battery refurbishment programs. Some manufacturers offer lifetime battery warranties, though these often come with usage restrictions. Meanwhile, a growing ecosystem of third-party solutions focuses on battery reconditioning and recycling.
The challenges of battery replacement costs underscore the importance of continued technological innovation in energy storage. As the EV market matures, advances in battery chemistry and manufacturing processes may help reduce replacement costs while extending battery lifespan.